Why doesn't H₄O²⁺ exist? Announcing the arrival of Valued Associate #679: Cesar Manara Planned maintenance scheduled April 23, 2019 at 23:30 UTC (7:30pm US/Eastern)What is the Ka of OH- and Kb of H3O+?Can H4O 2+ form?pKa differences and reactivitywhy there is no simple rule to predict the possible ionic charges of element in middle of periodic tableStability variation in heavy nucleiCan there be gaps in autoignition temperatures?Stability of alkenes by hyper conjugationstability of complexesWhen, how & why do atoms become stable. If they're always bonding, do pure elements even exist in the real world? What happens to unstable atoms?Polarisation of electron clouds by partially positively-charged hydrogen atomsNon existence of B3+ in solutionFabrication and/or Selection of a Single Crystal Ionic ConductorDoes tetraoxidovanadium (VO4+) ion exist?
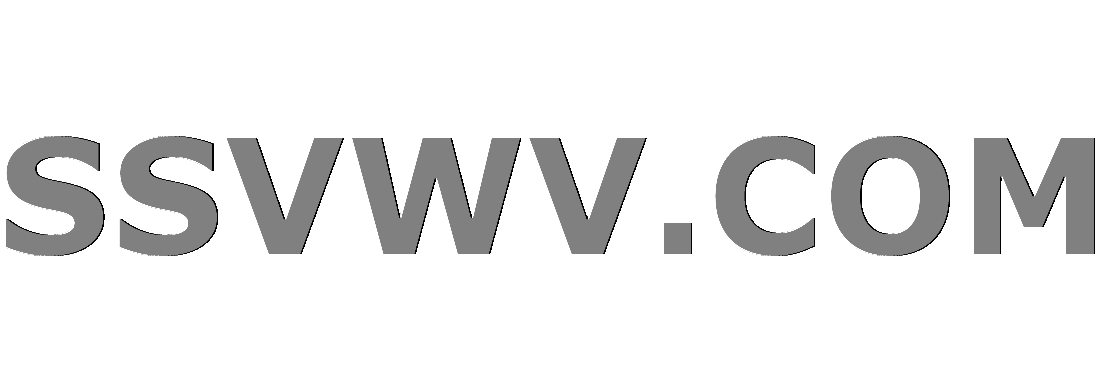
Multi tool use
Is it OK to use the testing sample to compare algorithms?
First paper to introduce the "principal-agent problem"
Does the Rock Gnome trait Artificer's Lore apply when you aren't proficient in History?
How to make triangles with rounded sides and corners? (squircle with 3 sides)
Simple Line in LaTeX Help!
How to resize main filesystem
Where did Ptolemy compare the Earth to the distance of fixed stars?
How to achieve cat-like agility?
What is "Lambda" in Heston's original paper on stochastic volatility models?
Why does BitLocker not use RSA?
Are there any irrational/transcendental numbers for which the distribution of decimal digits is not uniform?
One-one communication
Understanding piped commands in GNU/Linux
What was the last profitable war?
Marquee sign letters
How to infer difference of population proportion between two groups when proportion is small?
Vertical ranges of Column Plots in 12
Did any compiler fully use 80-bit floating point?
How to name indistinguishable henchmen in a screenplay?
How can I list files in reverse time order by a command and pass them as arguments to another command?
The test team as an enemy of development? And how can this be avoided?
Why complex landing gears are used instead of simple, reliable and light weight muscle wire or shape memory alloys?
Why is there so little support for joining EFTA in the British parliament?
malloc in main() or malloc in another function: allocating memory for a struct and its members
Why doesn't H₄O²⁺ exist?
Announcing the arrival of Valued Associate #679: Cesar Manara
Planned maintenance scheduled April 23, 2019 at 23:30 UTC (7:30pm US/Eastern)What is the Ka of OH- and Kb of H3O+?Can H4O 2+ form?pKa differences and reactivitywhy there is no simple rule to predict the possible ionic charges of element in middle of periodic tableStability variation in heavy nucleiCan there be gaps in autoignition temperatures?Stability of alkenes by hyper conjugationstability of complexesWhen, how & why do atoms become stable. If they're always bonding, do pure elements even exist in the real world? What happens to unstable atoms?Polarisation of electron clouds by partially positively-charged hydrogen atomsNon existence of B3+ in solutionFabrication and/or Selection of a Single Crystal Ionic ConductorDoes tetraoxidovanadium (VO4+) ion exist?
$begingroup$
I know this question has been asked previously but I cannot find a satisfactory explanation as to why is it so difficult for $ceH4O^2+$ to exist. There are explanations that it is so because of $+2$ charge, but if only that was the reason then existence of species like $ceSO4^2-$ should not have been possible.
So, what is exactly the reason that makes $ceH4O^2+$ so unstable?
ions stability
$endgroup$
|
show 5 more comments
$begingroup$
I know this question has been asked previously but I cannot find a satisfactory explanation as to why is it so difficult for $ceH4O^2+$ to exist. There are explanations that it is so because of $+2$ charge, but if only that was the reason then existence of species like $ceSO4^2-$ should not have been possible.
So, what is exactly the reason that makes $ceH4O^2+$ so unstable?
ions stability
$endgroup$
10
$begingroup$
Note that even $ceH3O+$ is questionable, as some sources prefer existence of $ceH9O4+ $, where each of 3 hydrogen atoms is bound to another $ceH2O$.
$endgroup$
– Poutnik
Apr 3 at 15:43
1
$begingroup$
Yes but isn't it kind of like hydration, where H2O molecules surround the ions , whereas in H3O+ a covalent bond exists between O and H?
$endgroup$
– Sanom Dane
Apr 3 at 15:48
3
$begingroup$
Well, it can be, it could be considered as coordination bonds of 3 H2O ligands. The idea was, that not only the naked $ceH+$ does not exist, but neither the naked $ceH3O+$. pubs.acs.org/doi/abs/10.1021/ic00126a005 A Crystalline [H9O4]+ Hydronium Ion Salt with a Weakly Coordinating Anion
$endgroup$
– Poutnik
Apr 3 at 15:53
2
$begingroup$
I do not doubt $ceH3O+$ exists, I have implied the liquid water context.
$endgroup$
– Poutnik
Apr 3 at 18:26
4
$begingroup$
Related question, though the asker may already be aware.
$endgroup$
– Nicolau Saker Neto
Apr 3 at 20:57
|
show 5 more comments
$begingroup$
I know this question has been asked previously but I cannot find a satisfactory explanation as to why is it so difficult for $ceH4O^2+$ to exist. There are explanations that it is so because of $+2$ charge, but if only that was the reason then existence of species like $ceSO4^2-$ should not have been possible.
So, what is exactly the reason that makes $ceH4O^2+$ so unstable?
ions stability
$endgroup$
I know this question has been asked previously but I cannot find a satisfactory explanation as to why is it so difficult for $ceH4O^2+$ to exist. There are explanations that it is so because of $+2$ charge, but if only that was the reason then existence of species like $ceSO4^2-$ should not have been possible.
So, what is exactly the reason that makes $ceH4O^2+$ so unstable?
ions stability
ions stability
edited Apr 4 at 13:43
Sanom Dane
asked Apr 3 at 15:39


Sanom DaneSanom Dane
412128
412128
10
$begingroup$
Note that even $ceH3O+$ is questionable, as some sources prefer existence of $ceH9O4+ $, where each of 3 hydrogen atoms is bound to another $ceH2O$.
$endgroup$
– Poutnik
Apr 3 at 15:43
1
$begingroup$
Yes but isn't it kind of like hydration, where H2O molecules surround the ions , whereas in H3O+ a covalent bond exists between O and H?
$endgroup$
– Sanom Dane
Apr 3 at 15:48
3
$begingroup$
Well, it can be, it could be considered as coordination bonds of 3 H2O ligands. The idea was, that not only the naked $ceH+$ does not exist, but neither the naked $ceH3O+$. pubs.acs.org/doi/abs/10.1021/ic00126a005 A Crystalline [H9O4]+ Hydronium Ion Salt with a Weakly Coordinating Anion
$endgroup$
– Poutnik
Apr 3 at 15:53
2
$begingroup$
I do not doubt $ceH3O+$ exists, I have implied the liquid water context.
$endgroup$
– Poutnik
Apr 3 at 18:26
4
$begingroup$
Related question, though the asker may already be aware.
$endgroup$
– Nicolau Saker Neto
Apr 3 at 20:57
|
show 5 more comments
10
$begingroup$
Note that even $ceH3O+$ is questionable, as some sources prefer existence of $ceH9O4+ $, where each of 3 hydrogen atoms is bound to another $ceH2O$.
$endgroup$
– Poutnik
Apr 3 at 15:43
1
$begingroup$
Yes but isn't it kind of like hydration, where H2O molecules surround the ions , whereas in H3O+ a covalent bond exists between O and H?
$endgroup$
– Sanom Dane
Apr 3 at 15:48
3
$begingroup$
Well, it can be, it could be considered as coordination bonds of 3 H2O ligands. The idea was, that not only the naked $ceH+$ does not exist, but neither the naked $ceH3O+$. pubs.acs.org/doi/abs/10.1021/ic00126a005 A Crystalline [H9O4]+ Hydronium Ion Salt with a Weakly Coordinating Anion
$endgroup$
– Poutnik
Apr 3 at 15:53
2
$begingroup$
I do not doubt $ceH3O+$ exists, I have implied the liquid water context.
$endgroup$
– Poutnik
Apr 3 at 18:26
4
$begingroup$
Related question, though the asker may already be aware.
$endgroup$
– Nicolau Saker Neto
Apr 3 at 20:57
10
10
$begingroup$
Note that even $ceH3O+$ is questionable, as some sources prefer existence of $ceH9O4+ $, where each of 3 hydrogen atoms is bound to another $ceH2O$.
$endgroup$
– Poutnik
Apr 3 at 15:43
$begingroup$
Note that even $ceH3O+$ is questionable, as some sources prefer existence of $ceH9O4+ $, where each of 3 hydrogen atoms is bound to another $ceH2O$.
$endgroup$
– Poutnik
Apr 3 at 15:43
1
1
$begingroup$
Yes but isn't it kind of like hydration, where H2O molecules surround the ions , whereas in H3O+ a covalent bond exists between O and H?
$endgroup$
– Sanom Dane
Apr 3 at 15:48
$begingroup$
Yes but isn't it kind of like hydration, where H2O molecules surround the ions , whereas in H3O+ a covalent bond exists between O and H?
$endgroup$
– Sanom Dane
Apr 3 at 15:48
3
3
$begingroup$
Well, it can be, it could be considered as coordination bonds of 3 H2O ligands. The idea was, that not only the naked $ceH+$ does not exist, but neither the naked $ceH3O+$. pubs.acs.org/doi/abs/10.1021/ic00126a005 A Crystalline [H9O4]+ Hydronium Ion Salt with a Weakly Coordinating Anion
$endgroup$
– Poutnik
Apr 3 at 15:53
$begingroup$
Well, it can be, it could be considered as coordination bonds of 3 H2O ligands. The idea was, that not only the naked $ceH+$ does not exist, but neither the naked $ceH3O+$. pubs.acs.org/doi/abs/10.1021/ic00126a005 A Crystalline [H9O4]+ Hydronium Ion Salt with a Weakly Coordinating Anion
$endgroup$
– Poutnik
Apr 3 at 15:53
2
2
$begingroup$
I do not doubt $ceH3O+$ exists, I have implied the liquid water context.
$endgroup$
– Poutnik
Apr 3 at 18:26
$begingroup$
I do not doubt $ceH3O+$ exists, I have implied the liquid water context.
$endgroup$
– Poutnik
Apr 3 at 18:26
4
4
$begingroup$
Related question, though the asker may already be aware.
$endgroup$
– Nicolau Saker Neto
Apr 3 at 20:57
$begingroup$
Related question, though the asker may already be aware.
$endgroup$
– Nicolau Saker Neto
Apr 3 at 20:57
|
show 5 more comments
3 Answers
3
active
oldest
votes
$begingroup$
I myself was always confused why $ceH3O^+$ is so well-known and yet almost nobody talks of $ceH4O^2+$. I mean, $ceH3O^+$ still has a lone pair, right? Why can't another proton just latch onto that? Adding to the confusion, $ceH4O^2+$ is very similar to $ceNH4+$, which again is extremely well-known. Even further, the methanium cation $ceCH5+$ exists (admittedly not something you'll find on a shelf), and that doesn't even have an available lone pair!
It is very useful to rephrase the question "why is $ceH4O^2+$ so rare?" into "why won't $ceH3O^+$ accept another proton?". Now we can think of this in terms of an acid-base reaction:
$$ceH3O^+ + H+ -> H4O^2+$$
Yes, that's right. In this reaction $ceH3O^+$ is the base, and $ceH^+$ is the acid. Because solvents can strongly influence the acidity of basicity of dissolved compounds, and because inclusion of solvent makes calculations tremendously more complicated, we will restrict ourselves to the gas phase (hence $ce(g)$ next to all the formulas). This means we will be talking about proton affinities.
Before we get to business, though, let's start with something more familiar:
$$ceH2O(g) + H+(g) -> H3O^+(g)$$
Because this is in the gas phase, we can visualise the process very simply. We start with a lone water molecule in a perfect vacuum. Then, from a very large distance away, a lone proton begins its approach. We can calculate the potential energy of the whole system as a function of the distance between the oxygen atom and the distant proton. We get a graph that looks something like this:
For convenience, we can set the potential energy of the system at 0 when the distance is infinite. At very large distances, the lone proton only very slightly tugs the electrons of the $ceH2O$ molecule, but they attract and the system is slightly stabilised. The attraction gets stronger as the lone proton approaches. However, there is also a repulsive interaction, between the lone proton and the nuclei of the other atoms in the $ceH2O$ molecule. At large distances, the attraction is stronger than the repulsion, but this flips around if the distance is too short. The happy medium is where the extra proton is close enough to dive into the molecule's electron cloud, but not close enough to experience severe repulsions with the other nuclei.
In short, a lone proton from infinity is attracted to a water molecule, and the potential energy decreases up to a critical value, the bond length. The amount of energy lost is the proton affinity: in this scenario, a mole of water molecules reacting with a mole of protons would release approximately $mathrm697 kJ mol^-1$ (values from this table). This reaction is highly exothermic
Alright, now for the next step:
$$ceH3O^+(g) + H+(g) -> H4O^2+(g)$$
This should be similar, right? Actually, no. There is a very important difference between this reaction and the previous one; the reagents now both have a net positive charge. This means there is now a strong additional repulsive force between the two. In fact, the graph above changes completely. Starting from zero potential at infinity, instead of a slow decrease in potential energy, the lone proton has to climb uphill, fighting a net electrostatic repulsion. However, even more interestingly, if the proton does manage to get close enough, the electron cloud can abruptly envelop the additional proton and create a net attraction. The resulting graph now looks more like this:
Very interestingly, the bottom of the "pocket" on the left of the graph (the potential well) can have a higher potential energy than if the lone proton was infinitely far away. This means the reaction is endothermic, but with enough effort, an extra proton can be pushed into the molecule, and it gets trapped in the pocket. Indeed, according to Olah et al., J. Am. Chem. Soc. 1986, 108 (5), pp 1032-1035, the formation of $ceH4O^2+$ in the gas phase was calculated to be endothermic by $mathrm248 kJ mol^-1$ (that is, the proton affinity of $ceH3O^+$ is $mathrm-248 kJ mol^-1$), but once formed, it has a barrier towards decomposition (the activation energy towards release of a proton) of $mathrm184 kJ mol^-1$ (the potential well has a maximum depth of $mathrm184 kJ mol^-1$).
Due to the fact that $ceH4O^2+$ was calculated to form a potential well, it can in principle exist. However, since it is the product of a highly endothermic reaction, unsurprisingly it is very hard to find. The reality in solution phase is more complicated, but its existence has been physically verified (if indirectly).
But why stop here? What about $ceH5O^3+$?
$$ceH4O^2+(g) + H+(g) -> H5O^3+(g)$$
I've run a rough calculation myself using computational chemistry software, and here it seems we really do reach a wall. It appears that $ceH5O^3+$ is an unbound system, which is to say that its potential energy curve has no pocket like the ones above. $ceH5O^3+$ could only ever be made transiently, and it would immediately spit out at least one proton. The reason here really is the massive amount of electrical repulsion, combined with the fact that the electron cloud can't reach out to the distance necessary to accommodate another atom.
You can make your own potential energy graphs here. Note how depending on the combination of parameters, the potential well can lie at negative potential energies (an exothermic reaction) or positive potential energies (an endothermic reaction). Alternatively, the pocket may not exist at all - these are the unbound systems.
EDIT: I've done some calculations of proton affinities/stabilities on several other simple molecules, for comparison. I do not claim the results to be quantitatively correct.
$$
beginarraylllll
textSpecies & ceCH4 & ceCH5+ & ceCH6^2+ & ceCH7^3+ & ceCH8^4+ \
textStable in gas phase? & textYes & textYes & textYes & textYes & textNo \
textApproximate proton affinity (mathrmkJ mol^-1) & 556 & -246 & -1020 & N/A & N/A \
endarray
$$
Notes:
Even without a lone pair, methane ($ceCH4$) protonates very exothermically in the gas phase. This is a testament to the enormous reactivity of a bare proton, and the huge difference it makes to not have push a proton into an already positively-charged ion.
For most of the seemingly hypercoordinate species in these tables (more than four bonds), the excess hydrogen atoms "pair up" such that it can be viewed as a $ceH2$ molecule binding sideways to the central atom. See the methanium link at the start.
$$
beginarraylllll
textSpecies & ceNH3 & ceNH4+ & ceNH5^2+ & ceNH6^3+ \
textStable in gas phase? & textYes & textYes & textYes & textNo \
textApproximate proton affinity (mathrmkJ mol^-1) & 896 & -410 & N/A & N/A \
endarray
$$
Notes:
Even though the first protonation is easier relative to $ceCH4$, the second one is harder. This is likely because increasing the electronegativity of the central atom makes the electron cloud "stiffer", and less accommodating to all those extra protons.
The $ceNH5^2+$ ion, unlike other ions listed here with more than four hydrogens, appears to be a true hypercoordinate species. Del Bene et al. indicate a five-coordinate square pyramidal structure with delocalized nitrogen-hydrogen bonds.
$$
beginarraylllll
textSpecies & ceH2O & ceH3O+ & ceH4O^2+ & ceH5O^3+ \
textStable in gas phase? & textYes & textYes & textYes & textNo \
textApproximate proton affinity (mathrmkJ mol^-1) & 722 & -236 & N/A & N/A \
endarray
$$
Notes:
- The first series which does not accommodate proton hypercoordination.
$ceH3O+$ is easier to protonate than $ceNH4+$, even though oxygen is more electronegative. This is because the $ceH4O^2+$ nicely accommodates all protons, while one of the protons in $ceNH5^2+$ has to fight for its space.
$$
beginarraylllll
textSpecies & ceHF & ceH2F+ & ceH3F^2+ & ceH4F^3+ \
textStable in gas phase? & textYes & textYes & textYes & textNo \
textApproximate proton affinity (mathrmkJ mol^-1) & 501 & -459 & N/A & N/A \
endarray
$$
Notes:
- Even though $ceH3F^2+$ still formally has a lone pair, its electron cloud is now so stiff that it cannot reach out to another proton even at normal bonding distance.
$$
beginarraylllll
textSpecies & ceNe & ceNeH+ & ceNeH2^2+ \
textStable in gas phase? & textYes & textYes & textNo \
textApproximate proton affinity (mathrmkJ mol^-1) & 204 & N/A & N/A \
endarray
$$
Notes:
$ceNe$ is a notoriously unreactive noble gas, but it too will react exothermically with a bare proton in the gas phase.- Depending on the definition of electronegativity used, it is possible to determine an electronegativity for $ceNe$, which turns out to be even higher than $ceF$. Accordingly, its electron cloud is even stiffer.
$$
beginarraylllll
textSpecies & ceH2S & ceH3S+ & ceH4S^2+ & ceH5S^3+ & ceH6S^4+ \
textStable in gas phase? & textYes & textYes & textYes & textYes & textNo \
textApproximate proton affinity (mathrmkJ mol^-1) & 752 & -121 & -1080 & N/A & N/A \
endarray
$$
Notes:
The lower electronegativity and larger size of $ceS$ means its electrons can reach out further and accommodate protons at a larger distance, while reducing repulsions between the nuclei. Thus, in the gas phase, $ceH2S$ is a stronger base than $ceH2O$. The situation is inverted in aqueous solution due to uniquely strong intermolecular interactions (hydrogen bonding) which are much more important for $ceH2O$.
$ceH3S+$ also has an endothermic proton affinity, but it is lower than for $ceH3O+$, and therefore $ceH4S^2+$ is easier to make. Accordingly, $ceH4S^2+$ has been detected in milder (though still superacidic!) conditions than $ceH4O^2+$.
The larger size and lower electronegativity of $ceS$ once again are shown to be important; the hypercoodinate $ceH5S^3+$ appears to exist, while the oxygen analogue doesn't.
$endgroup$
1
$begingroup$
@santimirandarp I don't have good graphing software on me right now, but I made something on Desmos. I also put a link to graphs that curious readers may play around with.
$endgroup$
– Nicolau Saker Neto
Apr 4 at 10:18
1
$begingroup$
Unstable by 248 kJ/mol! I smell a monopropellent.
$endgroup$
– Joshua
Apr 4 at 15:43
20
$begingroup$
I've rarely seen this level of investigation in an SE answer. This answer could be turned into a manuscript and published in a reputable journal.
$endgroup$
– WaterMolecule
Apr 4 at 18:42
3
$begingroup$
The "pairing up" of excess hydrogen atoms (beyond 4) may not be entirely accurate. This reference renders the NH5 cation as a square pyramid with multiple delocalized bonds.
$endgroup$
– Oscar Lanzi
Apr 4 at 22:11
1
$begingroup$
@OscarLanzi Huh, interestingly enough that's also what my calculations found, and I overlooked it. It seems to be the only truly hypercoordinate structure out of all the ones I calculated. I was planning on linking the geometries for each structure, but I'll have to do it later. Thanks for the tip, though!
$endgroup$
– Nicolau Saker Neto
Apr 4 at 22:19
|
show 2 more comments
$begingroup$
In chemistry ask "why" only after you ask "if".
Given a sufficiently strong superacidic medium, $ceH3O^+$ can be protonated to $ceH4O^2+$. Evidence for this reaction, by studying isotopic exchange in a $ceHF + SbF5 +SO2$ solvent, is given here.
$ceH4O^2+$ is, of course, a powerful protic acid, and it would be leveled to something weaker, meaning $ceH3O^+$ and maybe other products, except in superacids as in the above reference.
A subtle difference between $ceH4O^2+$ and $ceSO4^2-$ is the presence of atoms with odd atomic number in the former. An odd atomic number means an odd number of protons, so $ceH4O^2+$ can split off or transfer a component ($ceH^+$) having a single charge and an odd number of odd-proton atoms, without having to break up any electron pairs (no need to form radicals). Hence, unless the environment is suffuciently acidic/nonbasic, this ion could break up relatively easily to singly charged products. $ceSO4^2-$ lacks this option; as this ion has only even atomic number atoms it cannot break up into singly charged species without the unfavorable formation of radicals.
We see a similar phenomenon in the autoionization of solvents like water, ammonia or even dinitrogen tetroxide (p. 217). Solvents are more likely to autoionize if they have odd atomic number atoms (like hydrogen or nitrogen) with which singly charged, nonradical species can form.
$endgroup$
2
$begingroup$
Good point. If you can't break a molecule without making radicals or moving more than one atom (or subunit), you have a good chance it's at least metastable.
$endgroup$
– Karl
Apr 4 at 20:22
add a comment |
$begingroup$
$ceH4O^2+$ would be much smaller than $ceSO4^2-$. The charge density is too high much higher.
The oxygen in water already becomes electron-deficient when it forms $ceH3O+$. In your (not completely, see below) hypothetical $ceH4O^2+$, the additional proton barely finds any electron density to make a covalent-ish bond with.
Whereas for the sulfate ion, you just have to distribute some extra charge over a relatively large molecule.
So $ceH4O^2+$ exists, at least in theory, but it is extremely unstable. It's properties have been detailed (with links to scientific papers) here: What is the Ka of OH- and Kb of H3O+?
$endgroup$
3
$begingroup$
It is quite a valid species, OP is just wondering why it's very difficult to make, even transiently.
$endgroup$
– Mithoron
Apr 3 at 21:25
1
$begingroup$
@Mithoron Are you implying that this particle can exist, at least theoretically and with a very short lifetime, in a bound state? I find that hard to believe, unless of course you have some evidence? (Ok, found it under the link you gave above pubs.acs.org/doi/pdf/10.1021/ja00265a031)
$endgroup$
– Karl
Apr 3 at 21:50
1
$begingroup$
Yeah, it seems it's repulsive, but metastable.
$endgroup$
– Mithoron
Apr 3 at 22:17
add a comment |
Your Answer
StackExchange.ready(function()
var channelOptions =
tags: "".split(" "),
id: "431"
;
initTagRenderer("".split(" "), "".split(" "), channelOptions);
StackExchange.using("externalEditor", function()
// Have to fire editor after snippets, if snippets enabled
if (StackExchange.settings.snippets.snippetsEnabled)
StackExchange.using("snippets", function()
createEditor();
);
else
createEditor();
);
function createEditor()
StackExchange.prepareEditor(
heartbeatType: 'answer',
autoActivateHeartbeat: false,
convertImagesToLinks: false,
noModals: true,
showLowRepImageUploadWarning: true,
reputationToPostImages: null,
bindNavPrevention: true,
postfix: "",
imageUploader:
brandingHtml: "Powered by u003ca class="icon-imgur-white" href="https://imgur.com/"u003eu003c/au003e",
contentPolicyHtml: "User contributions licensed under u003ca href="https://creativecommons.org/licenses/by-sa/3.0/"u003ecc by-sa 3.0 with attribution requiredu003c/au003e u003ca href="https://stackoverflow.com/legal/content-policy"u003e(content policy)u003c/au003e",
allowUrls: true
,
onDemand: true,
discardSelector: ".discard-answer"
,immediatelyShowMarkdownHelp:true
);
);
Sign up or log in
StackExchange.ready(function ()
StackExchange.helpers.onClickDraftSave('#login-link');
);
Sign up using Google
Sign up using Facebook
Sign up using Email and Password
Post as a guest
Required, but never shown
StackExchange.ready(
function ()
StackExchange.openid.initPostLogin('.new-post-login', 'https%3a%2f%2fchemistry.stackexchange.com%2fquestions%2f112087%2fwhy-doesnt-h%25e2%2582%2584o%25c2%25b2%25e2%2581%25ba-exist%23new-answer', 'question_page');
);
Post as a guest
Required, but never shown
3 Answers
3
active
oldest
votes
3 Answers
3
active
oldest
votes
active
oldest
votes
active
oldest
votes
$begingroup$
I myself was always confused why $ceH3O^+$ is so well-known and yet almost nobody talks of $ceH4O^2+$. I mean, $ceH3O^+$ still has a lone pair, right? Why can't another proton just latch onto that? Adding to the confusion, $ceH4O^2+$ is very similar to $ceNH4+$, which again is extremely well-known. Even further, the methanium cation $ceCH5+$ exists (admittedly not something you'll find on a shelf), and that doesn't even have an available lone pair!
It is very useful to rephrase the question "why is $ceH4O^2+$ so rare?" into "why won't $ceH3O^+$ accept another proton?". Now we can think of this in terms of an acid-base reaction:
$$ceH3O^+ + H+ -> H4O^2+$$
Yes, that's right. In this reaction $ceH3O^+$ is the base, and $ceH^+$ is the acid. Because solvents can strongly influence the acidity of basicity of dissolved compounds, and because inclusion of solvent makes calculations tremendously more complicated, we will restrict ourselves to the gas phase (hence $ce(g)$ next to all the formulas). This means we will be talking about proton affinities.
Before we get to business, though, let's start with something more familiar:
$$ceH2O(g) + H+(g) -> H3O^+(g)$$
Because this is in the gas phase, we can visualise the process very simply. We start with a lone water molecule in a perfect vacuum. Then, from a very large distance away, a lone proton begins its approach. We can calculate the potential energy of the whole system as a function of the distance between the oxygen atom and the distant proton. We get a graph that looks something like this:
For convenience, we can set the potential energy of the system at 0 when the distance is infinite. At very large distances, the lone proton only very slightly tugs the electrons of the $ceH2O$ molecule, but they attract and the system is slightly stabilised. The attraction gets stronger as the lone proton approaches. However, there is also a repulsive interaction, between the lone proton and the nuclei of the other atoms in the $ceH2O$ molecule. At large distances, the attraction is stronger than the repulsion, but this flips around if the distance is too short. The happy medium is where the extra proton is close enough to dive into the molecule's electron cloud, but not close enough to experience severe repulsions with the other nuclei.
In short, a lone proton from infinity is attracted to a water molecule, and the potential energy decreases up to a critical value, the bond length. The amount of energy lost is the proton affinity: in this scenario, a mole of water molecules reacting with a mole of protons would release approximately $mathrm697 kJ mol^-1$ (values from this table). This reaction is highly exothermic
Alright, now for the next step:
$$ceH3O^+(g) + H+(g) -> H4O^2+(g)$$
This should be similar, right? Actually, no. There is a very important difference between this reaction and the previous one; the reagents now both have a net positive charge. This means there is now a strong additional repulsive force between the two. In fact, the graph above changes completely. Starting from zero potential at infinity, instead of a slow decrease in potential energy, the lone proton has to climb uphill, fighting a net electrostatic repulsion. However, even more interestingly, if the proton does manage to get close enough, the electron cloud can abruptly envelop the additional proton and create a net attraction. The resulting graph now looks more like this:
Very interestingly, the bottom of the "pocket" on the left of the graph (the potential well) can have a higher potential energy than if the lone proton was infinitely far away. This means the reaction is endothermic, but with enough effort, an extra proton can be pushed into the molecule, and it gets trapped in the pocket. Indeed, according to Olah et al., J. Am. Chem. Soc. 1986, 108 (5), pp 1032-1035, the formation of $ceH4O^2+$ in the gas phase was calculated to be endothermic by $mathrm248 kJ mol^-1$ (that is, the proton affinity of $ceH3O^+$ is $mathrm-248 kJ mol^-1$), but once formed, it has a barrier towards decomposition (the activation energy towards release of a proton) of $mathrm184 kJ mol^-1$ (the potential well has a maximum depth of $mathrm184 kJ mol^-1$).
Due to the fact that $ceH4O^2+$ was calculated to form a potential well, it can in principle exist. However, since it is the product of a highly endothermic reaction, unsurprisingly it is very hard to find. The reality in solution phase is more complicated, but its existence has been physically verified (if indirectly).
But why stop here? What about $ceH5O^3+$?
$$ceH4O^2+(g) + H+(g) -> H5O^3+(g)$$
I've run a rough calculation myself using computational chemistry software, and here it seems we really do reach a wall. It appears that $ceH5O^3+$ is an unbound system, which is to say that its potential energy curve has no pocket like the ones above. $ceH5O^3+$ could only ever be made transiently, and it would immediately spit out at least one proton. The reason here really is the massive amount of electrical repulsion, combined with the fact that the electron cloud can't reach out to the distance necessary to accommodate another atom.
You can make your own potential energy graphs here. Note how depending on the combination of parameters, the potential well can lie at negative potential energies (an exothermic reaction) or positive potential energies (an endothermic reaction). Alternatively, the pocket may not exist at all - these are the unbound systems.
EDIT: I've done some calculations of proton affinities/stabilities on several other simple molecules, for comparison. I do not claim the results to be quantitatively correct.
$$
beginarraylllll
textSpecies & ceCH4 & ceCH5+ & ceCH6^2+ & ceCH7^3+ & ceCH8^4+ \
textStable in gas phase? & textYes & textYes & textYes & textYes & textNo \
textApproximate proton affinity (mathrmkJ mol^-1) & 556 & -246 & -1020 & N/A & N/A \
endarray
$$
Notes:
Even without a lone pair, methane ($ceCH4$) protonates very exothermically in the gas phase. This is a testament to the enormous reactivity of a bare proton, and the huge difference it makes to not have push a proton into an already positively-charged ion.
For most of the seemingly hypercoordinate species in these tables (more than four bonds), the excess hydrogen atoms "pair up" such that it can be viewed as a $ceH2$ molecule binding sideways to the central atom. See the methanium link at the start.
$$
beginarraylllll
textSpecies & ceNH3 & ceNH4+ & ceNH5^2+ & ceNH6^3+ \
textStable in gas phase? & textYes & textYes & textYes & textNo \
textApproximate proton affinity (mathrmkJ mol^-1) & 896 & -410 & N/A & N/A \
endarray
$$
Notes:
Even though the first protonation is easier relative to $ceCH4$, the second one is harder. This is likely because increasing the electronegativity of the central atom makes the electron cloud "stiffer", and less accommodating to all those extra protons.
The $ceNH5^2+$ ion, unlike other ions listed here with more than four hydrogens, appears to be a true hypercoordinate species. Del Bene et al. indicate a five-coordinate square pyramidal structure with delocalized nitrogen-hydrogen bonds.
$$
beginarraylllll
textSpecies & ceH2O & ceH3O+ & ceH4O^2+ & ceH5O^3+ \
textStable in gas phase? & textYes & textYes & textYes & textNo \
textApproximate proton affinity (mathrmkJ mol^-1) & 722 & -236 & N/A & N/A \
endarray
$$
Notes:
- The first series which does not accommodate proton hypercoordination.
$ceH3O+$ is easier to protonate than $ceNH4+$, even though oxygen is more electronegative. This is because the $ceH4O^2+$ nicely accommodates all protons, while one of the protons in $ceNH5^2+$ has to fight for its space.
$$
beginarraylllll
textSpecies & ceHF & ceH2F+ & ceH3F^2+ & ceH4F^3+ \
textStable in gas phase? & textYes & textYes & textYes & textNo \
textApproximate proton affinity (mathrmkJ mol^-1) & 501 & -459 & N/A & N/A \
endarray
$$
Notes:
- Even though $ceH3F^2+$ still formally has a lone pair, its electron cloud is now so stiff that it cannot reach out to another proton even at normal bonding distance.
$$
beginarraylllll
textSpecies & ceNe & ceNeH+ & ceNeH2^2+ \
textStable in gas phase? & textYes & textYes & textNo \
textApproximate proton affinity (mathrmkJ mol^-1) & 204 & N/A & N/A \
endarray
$$
Notes:
$ceNe$ is a notoriously unreactive noble gas, but it too will react exothermically with a bare proton in the gas phase.- Depending on the definition of electronegativity used, it is possible to determine an electronegativity for $ceNe$, which turns out to be even higher than $ceF$. Accordingly, its electron cloud is even stiffer.
$$
beginarraylllll
textSpecies & ceH2S & ceH3S+ & ceH4S^2+ & ceH5S^3+ & ceH6S^4+ \
textStable in gas phase? & textYes & textYes & textYes & textYes & textNo \
textApproximate proton affinity (mathrmkJ mol^-1) & 752 & -121 & -1080 & N/A & N/A \
endarray
$$
Notes:
The lower electronegativity and larger size of $ceS$ means its electrons can reach out further and accommodate protons at a larger distance, while reducing repulsions between the nuclei. Thus, in the gas phase, $ceH2S$ is a stronger base than $ceH2O$. The situation is inverted in aqueous solution due to uniquely strong intermolecular interactions (hydrogen bonding) which are much more important for $ceH2O$.
$ceH3S+$ also has an endothermic proton affinity, but it is lower than for $ceH3O+$, and therefore $ceH4S^2+$ is easier to make. Accordingly, $ceH4S^2+$ has been detected in milder (though still superacidic!) conditions than $ceH4O^2+$.
The larger size and lower electronegativity of $ceS$ once again are shown to be important; the hypercoodinate $ceH5S^3+$ appears to exist, while the oxygen analogue doesn't.
$endgroup$
1
$begingroup$
@santimirandarp I don't have good graphing software on me right now, but I made something on Desmos. I also put a link to graphs that curious readers may play around with.
$endgroup$
– Nicolau Saker Neto
Apr 4 at 10:18
1
$begingroup$
Unstable by 248 kJ/mol! I smell a monopropellent.
$endgroup$
– Joshua
Apr 4 at 15:43
20
$begingroup$
I've rarely seen this level of investigation in an SE answer. This answer could be turned into a manuscript and published in a reputable journal.
$endgroup$
– WaterMolecule
Apr 4 at 18:42
3
$begingroup$
The "pairing up" of excess hydrogen atoms (beyond 4) may not be entirely accurate. This reference renders the NH5 cation as a square pyramid with multiple delocalized bonds.
$endgroup$
– Oscar Lanzi
Apr 4 at 22:11
1
$begingroup$
@OscarLanzi Huh, interestingly enough that's also what my calculations found, and I overlooked it. It seems to be the only truly hypercoordinate structure out of all the ones I calculated. I was planning on linking the geometries for each structure, but I'll have to do it later. Thanks for the tip, though!
$endgroup$
– Nicolau Saker Neto
Apr 4 at 22:19
|
show 2 more comments
$begingroup$
I myself was always confused why $ceH3O^+$ is so well-known and yet almost nobody talks of $ceH4O^2+$. I mean, $ceH3O^+$ still has a lone pair, right? Why can't another proton just latch onto that? Adding to the confusion, $ceH4O^2+$ is very similar to $ceNH4+$, which again is extremely well-known. Even further, the methanium cation $ceCH5+$ exists (admittedly not something you'll find on a shelf), and that doesn't even have an available lone pair!
It is very useful to rephrase the question "why is $ceH4O^2+$ so rare?" into "why won't $ceH3O^+$ accept another proton?". Now we can think of this in terms of an acid-base reaction:
$$ceH3O^+ + H+ -> H4O^2+$$
Yes, that's right. In this reaction $ceH3O^+$ is the base, and $ceH^+$ is the acid. Because solvents can strongly influence the acidity of basicity of dissolved compounds, and because inclusion of solvent makes calculations tremendously more complicated, we will restrict ourselves to the gas phase (hence $ce(g)$ next to all the formulas). This means we will be talking about proton affinities.
Before we get to business, though, let's start with something more familiar:
$$ceH2O(g) + H+(g) -> H3O^+(g)$$
Because this is in the gas phase, we can visualise the process very simply. We start with a lone water molecule in a perfect vacuum. Then, from a very large distance away, a lone proton begins its approach. We can calculate the potential energy of the whole system as a function of the distance between the oxygen atom and the distant proton. We get a graph that looks something like this:
For convenience, we can set the potential energy of the system at 0 when the distance is infinite. At very large distances, the lone proton only very slightly tugs the electrons of the $ceH2O$ molecule, but they attract and the system is slightly stabilised. The attraction gets stronger as the lone proton approaches. However, there is also a repulsive interaction, between the lone proton and the nuclei of the other atoms in the $ceH2O$ molecule. At large distances, the attraction is stronger than the repulsion, but this flips around if the distance is too short. The happy medium is where the extra proton is close enough to dive into the molecule's electron cloud, but not close enough to experience severe repulsions with the other nuclei.
In short, a lone proton from infinity is attracted to a water molecule, and the potential energy decreases up to a critical value, the bond length. The amount of energy lost is the proton affinity: in this scenario, a mole of water molecules reacting with a mole of protons would release approximately $mathrm697 kJ mol^-1$ (values from this table). This reaction is highly exothermic
Alright, now for the next step:
$$ceH3O^+(g) + H+(g) -> H4O^2+(g)$$
This should be similar, right? Actually, no. There is a very important difference between this reaction and the previous one; the reagents now both have a net positive charge. This means there is now a strong additional repulsive force between the two. In fact, the graph above changes completely. Starting from zero potential at infinity, instead of a slow decrease in potential energy, the lone proton has to climb uphill, fighting a net electrostatic repulsion. However, even more interestingly, if the proton does manage to get close enough, the electron cloud can abruptly envelop the additional proton and create a net attraction. The resulting graph now looks more like this:
Very interestingly, the bottom of the "pocket" on the left of the graph (the potential well) can have a higher potential energy than if the lone proton was infinitely far away. This means the reaction is endothermic, but with enough effort, an extra proton can be pushed into the molecule, and it gets trapped in the pocket. Indeed, according to Olah et al., J. Am. Chem. Soc. 1986, 108 (5), pp 1032-1035, the formation of $ceH4O^2+$ in the gas phase was calculated to be endothermic by $mathrm248 kJ mol^-1$ (that is, the proton affinity of $ceH3O^+$ is $mathrm-248 kJ mol^-1$), but once formed, it has a barrier towards decomposition (the activation energy towards release of a proton) of $mathrm184 kJ mol^-1$ (the potential well has a maximum depth of $mathrm184 kJ mol^-1$).
Due to the fact that $ceH4O^2+$ was calculated to form a potential well, it can in principle exist. However, since it is the product of a highly endothermic reaction, unsurprisingly it is very hard to find. The reality in solution phase is more complicated, but its existence has been physically verified (if indirectly).
But why stop here? What about $ceH5O^3+$?
$$ceH4O^2+(g) + H+(g) -> H5O^3+(g)$$
I've run a rough calculation myself using computational chemistry software, and here it seems we really do reach a wall. It appears that $ceH5O^3+$ is an unbound system, which is to say that its potential energy curve has no pocket like the ones above. $ceH5O^3+$ could only ever be made transiently, and it would immediately spit out at least one proton. The reason here really is the massive amount of electrical repulsion, combined with the fact that the electron cloud can't reach out to the distance necessary to accommodate another atom.
You can make your own potential energy graphs here. Note how depending on the combination of parameters, the potential well can lie at negative potential energies (an exothermic reaction) or positive potential energies (an endothermic reaction). Alternatively, the pocket may not exist at all - these are the unbound systems.
EDIT: I've done some calculations of proton affinities/stabilities on several other simple molecules, for comparison. I do not claim the results to be quantitatively correct.
$$
beginarraylllll
textSpecies & ceCH4 & ceCH5+ & ceCH6^2+ & ceCH7^3+ & ceCH8^4+ \
textStable in gas phase? & textYes & textYes & textYes & textYes & textNo \
textApproximate proton affinity (mathrmkJ mol^-1) & 556 & -246 & -1020 & N/A & N/A \
endarray
$$
Notes:
Even without a lone pair, methane ($ceCH4$) protonates very exothermically in the gas phase. This is a testament to the enormous reactivity of a bare proton, and the huge difference it makes to not have push a proton into an already positively-charged ion.
For most of the seemingly hypercoordinate species in these tables (more than four bonds), the excess hydrogen atoms "pair up" such that it can be viewed as a $ceH2$ molecule binding sideways to the central atom. See the methanium link at the start.
$$
beginarraylllll
textSpecies & ceNH3 & ceNH4+ & ceNH5^2+ & ceNH6^3+ \
textStable in gas phase? & textYes & textYes & textYes & textNo \
textApproximate proton affinity (mathrmkJ mol^-1) & 896 & -410 & N/A & N/A \
endarray
$$
Notes:
Even though the first protonation is easier relative to $ceCH4$, the second one is harder. This is likely because increasing the electronegativity of the central atom makes the electron cloud "stiffer", and less accommodating to all those extra protons.
The $ceNH5^2+$ ion, unlike other ions listed here with more than four hydrogens, appears to be a true hypercoordinate species. Del Bene et al. indicate a five-coordinate square pyramidal structure with delocalized nitrogen-hydrogen bonds.
$$
beginarraylllll
textSpecies & ceH2O & ceH3O+ & ceH4O^2+ & ceH5O^3+ \
textStable in gas phase? & textYes & textYes & textYes & textNo \
textApproximate proton affinity (mathrmkJ mol^-1) & 722 & -236 & N/A & N/A \
endarray
$$
Notes:
- The first series which does not accommodate proton hypercoordination.
$ceH3O+$ is easier to protonate than $ceNH4+$, even though oxygen is more electronegative. This is because the $ceH4O^2+$ nicely accommodates all protons, while one of the protons in $ceNH5^2+$ has to fight for its space.
$$
beginarraylllll
textSpecies & ceHF & ceH2F+ & ceH3F^2+ & ceH4F^3+ \
textStable in gas phase? & textYes & textYes & textYes & textNo \
textApproximate proton affinity (mathrmkJ mol^-1) & 501 & -459 & N/A & N/A \
endarray
$$
Notes:
- Even though $ceH3F^2+$ still formally has a lone pair, its electron cloud is now so stiff that it cannot reach out to another proton even at normal bonding distance.
$$
beginarraylllll
textSpecies & ceNe & ceNeH+ & ceNeH2^2+ \
textStable in gas phase? & textYes & textYes & textNo \
textApproximate proton affinity (mathrmkJ mol^-1) & 204 & N/A & N/A \
endarray
$$
Notes:
$ceNe$ is a notoriously unreactive noble gas, but it too will react exothermically with a bare proton in the gas phase.- Depending on the definition of electronegativity used, it is possible to determine an electronegativity for $ceNe$, which turns out to be even higher than $ceF$. Accordingly, its electron cloud is even stiffer.
$$
beginarraylllll
textSpecies & ceH2S & ceH3S+ & ceH4S^2+ & ceH5S^3+ & ceH6S^4+ \
textStable in gas phase? & textYes & textYes & textYes & textYes & textNo \
textApproximate proton affinity (mathrmkJ mol^-1) & 752 & -121 & -1080 & N/A & N/A \
endarray
$$
Notes:
The lower electronegativity and larger size of $ceS$ means its electrons can reach out further and accommodate protons at a larger distance, while reducing repulsions between the nuclei. Thus, in the gas phase, $ceH2S$ is a stronger base than $ceH2O$. The situation is inverted in aqueous solution due to uniquely strong intermolecular interactions (hydrogen bonding) which are much more important for $ceH2O$.
$ceH3S+$ also has an endothermic proton affinity, but it is lower than for $ceH3O+$, and therefore $ceH4S^2+$ is easier to make. Accordingly, $ceH4S^2+$ has been detected in milder (though still superacidic!) conditions than $ceH4O^2+$.
The larger size and lower electronegativity of $ceS$ once again are shown to be important; the hypercoodinate $ceH5S^3+$ appears to exist, while the oxygen analogue doesn't.
$endgroup$
1
$begingroup$
@santimirandarp I don't have good graphing software on me right now, but I made something on Desmos. I also put a link to graphs that curious readers may play around with.
$endgroup$
– Nicolau Saker Neto
Apr 4 at 10:18
1
$begingroup$
Unstable by 248 kJ/mol! I smell a monopropellent.
$endgroup$
– Joshua
Apr 4 at 15:43
20
$begingroup$
I've rarely seen this level of investigation in an SE answer. This answer could be turned into a manuscript and published in a reputable journal.
$endgroup$
– WaterMolecule
Apr 4 at 18:42
3
$begingroup$
The "pairing up" of excess hydrogen atoms (beyond 4) may not be entirely accurate. This reference renders the NH5 cation as a square pyramid with multiple delocalized bonds.
$endgroup$
– Oscar Lanzi
Apr 4 at 22:11
1
$begingroup$
@OscarLanzi Huh, interestingly enough that's also what my calculations found, and I overlooked it. It seems to be the only truly hypercoordinate structure out of all the ones I calculated. I was planning on linking the geometries for each structure, but I'll have to do it later. Thanks for the tip, though!
$endgroup$
– Nicolau Saker Neto
Apr 4 at 22:19
|
show 2 more comments
$begingroup$
I myself was always confused why $ceH3O^+$ is so well-known and yet almost nobody talks of $ceH4O^2+$. I mean, $ceH3O^+$ still has a lone pair, right? Why can't another proton just latch onto that? Adding to the confusion, $ceH4O^2+$ is very similar to $ceNH4+$, which again is extremely well-known. Even further, the methanium cation $ceCH5+$ exists (admittedly not something you'll find on a shelf), and that doesn't even have an available lone pair!
It is very useful to rephrase the question "why is $ceH4O^2+$ so rare?" into "why won't $ceH3O^+$ accept another proton?". Now we can think of this in terms of an acid-base reaction:
$$ceH3O^+ + H+ -> H4O^2+$$
Yes, that's right. In this reaction $ceH3O^+$ is the base, and $ceH^+$ is the acid. Because solvents can strongly influence the acidity of basicity of dissolved compounds, and because inclusion of solvent makes calculations tremendously more complicated, we will restrict ourselves to the gas phase (hence $ce(g)$ next to all the formulas). This means we will be talking about proton affinities.
Before we get to business, though, let's start with something more familiar:
$$ceH2O(g) + H+(g) -> H3O^+(g)$$
Because this is in the gas phase, we can visualise the process very simply. We start with a lone water molecule in a perfect vacuum. Then, from a very large distance away, a lone proton begins its approach. We can calculate the potential energy of the whole system as a function of the distance between the oxygen atom and the distant proton. We get a graph that looks something like this:
For convenience, we can set the potential energy of the system at 0 when the distance is infinite. At very large distances, the lone proton only very slightly tugs the electrons of the $ceH2O$ molecule, but they attract and the system is slightly stabilised. The attraction gets stronger as the lone proton approaches. However, there is also a repulsive interaction, between the lone proton and the nuclei of the other atoms in the $ceH2O$ molecule. At large distances, the attraction is stronger than the repulsion, but this flips around if the distance is too short. The happy medium is where the extra proton is close enough to dive into the molecule's electron cloud, but not close enough to experience severe repulsions with the other nuclei.
In short, a lone proton from infinity is attracted to a water molecule, and the potential energy decreases up to a critical value, the bond length. The amount of energy lost is the proton affinity: in this scenario, a mole of water molecules reacting with a mole of protons would release approximately $mathrm697 kJ mol^-1$ (values from this table). This reaction is highly exothermic
Alright, now for the next step:
$$ceH3O^+(g) + H+(g) -> H4O^2+(g)$$
This should be similar, right? Actually, no. There is a very important difference between this reaction and the previous one; the reagents now both have a net positive charge. This means there is now a strong additional repulsive force between the two. In fact, the graph above changes completely. Starting from zero potential at infinity, instead of a slow decrease in potential energy, the lone proton has to climb uphill, fighting a net electrostatic repulsion. However, even more interestingly, if the proton does manage to get close enough, the electron cloud can abruptly envelop the additional proton and create a net attraction. The resulting graph now looks more like this:
Very interestingly, the bottom of the "pocket" on the left of the graph (the potential well) can have a higher potential energy than if the lone proton was infinitely far away. This means the reaction is endothermic, but with enough effort, an extra proton can be pushed into the molecule, and it gets trapped in the pocket. Indeed, according to Olah et al., J. Am. Chem. Soc. 1986, 108 (5), pp 1032-1035, the formation of $ceH4O^2+$ in the gas phase was calculated to be endothermic by $mathrm248 kJ mol^-1$ (that is, the proton affinity of $ceH3O^+$ is $mathrm-248 kJ mol^-1$), but once formed, it has a barrier towards decomposition (the activation energy towards release of a proton) of $mathrm184 kJ mol^-1$ (the potential well has a maximum depth of $mathrm184 kJ mol^-1$).
Due to the fact that $ceH4O^2+$ was calculated to form a potential well, it can in principle exist. However, since it is the product of a highly endothermic reaction, unsurprisingly it is very hard to find. The reality in solution phase is more complicated, but its existence has been physically verified (if indirectly).
But why stop here? What about $ceH5O^3+$?
$$ceH4O^2+(g) + H+(g) -> H5O^3+(g)$$
I've run a rough calculation myself using computational chemistry software, and here it seems we really do reach a wall. It appears that $ceH5O^3+$ is an unbound system, which is to say that its potential energy curve has no pocket like the ones above. $ceH5O^3+$ could only ever be made transiently, and it would immediately spit out at least one proton. The reason here really is the massive amount of electrical repulsion, combined with the fact that the electron cloud can't reach out to the distance necessary to accommodate another atom.
You can make your own potential energy graphs here. Note how depending on the combination of parameters, the potential well can lie at negative potential energies (an exothermic reaction) or positive potential energies (an endothermic reaction). Alternatively, the pocket may not exist at all - these are the unbound systems.
EDIT: I've done some calculations of proton affinities/stabilities on several other simple molecules, for comparison. I do not claim the results to be quantitatively correct.
$$
beginarraylllll
textSpecies & ceCH4 & ceCH5+ & ceCH6^2+ & ceCH7^3+ & ceCH8^4+ \
textStable in gas phase? & textYes & textYes & textYes & textYes & textNo \
textApproximate proton affinity (mathrmkJ mol^-1) & 556 & -246 & -1020 & N/A & N/A \
endarray
$$
Notes:
Even without a lone pair, methane ($ceCH4$) protonates very exothermically in the gas phase. This is a testament to the enormous reactivity of a bare proton, and the huge difference it makes to not have push a proton into an already positively-charged ion.
For most of the seemingly hypercoordinate species in these tables (more than four bonds), the excess hydrogen atoms "pair up" such that it can be viewed as a $ceH2$ molecule binding sideways to the central atom. See the methanium link at the start.
$$
beginarraylllll
textSpecies & ceNH3 & ceNH4+ & ceNH5^2+ & ceNH6^3+ \
textStable in gas phase? & textYes & textYes & textYes & textNo \
textApproximate proton affinity (mathrmkJ mol^-1) & 896 & -410 & N/A & N/A \
endarray
$$
Notes:
Even though the first protonation is easier relative to $ceCH4$, the second one is harder. This is likely because increasing the electronegativity of the central atom makes the electron cloud "stiffer", and less accommodating to all those extra protons.
The $ceNH5^2+$ ion, unlike other ions listed here with more than four hydrogens, appears to be a true hypercoordinate species. Del Bene et al. indicate a five-coordinate square pyramidal structure with delocalized nitrogen-hydrogen bonds.
$$
beginarraylllll
textSpecies & ceH2O & ceH3O+ & ceH4O^2+ & ceH5O^3+ \
textStable in gas phase? & textYes & textYes & textYes & textNo \
textApproximate proton affinity (mathrmkJ mol^-1) & 722 & -236 & N/A & N/A \
endarray
$$
Notes:
- The first series which does not accommodate proton hypercoordination.
$ceH3O+$ is easier to protonate than $ceNH4+$, even though oxygen is more electronegative. This is because the $ceH4O^2+$ nicely accommodates all protons, while one of the protons in $ceNH5^2+$ has to fight for its space.
$$
beginarraylllll
textSpecies & ceHF & ceH2F+ & ceH3F^2+ & ceH4F^3+ \
textStable in gas phase? & textYes & textYes & textYes & textNo \
textApproximate proton affinity (mathrmkJ mol^-1) & 501 & -459 & N/A & N/A \
endarray
$$
Notes:
- Even though $ceH3F^2+$ still formally has a lone pair, its electron cloud is now so stiff that it cannot reach out to another proton even at normal bonding distance.
$$
beginarraylllll
textSpecies & ceNe & ceNeH+ & ceNeH2^2+ \
textStable in gas phase? & textYes & textYes & textNo \
textApproximate proton affinity (mathrmkJ mol^-1) & 204 & N/A & N/A \
endarray
$$
Notes:
$ceNe$ is a notoriously unreactive noble gas, but it too will react exothermically with a bare proton in the gas phase.- Depending on the definition of electronegativity used, it is possible to determine an electronegativity for $ceNe$, which turns out to be even higher than $ceF$. Accordingly, its electron cloud is even stiffer.
$$
beginarraylllll
textSpecies & ceH2S & ceH3S+ & ceH4S^2+ & ceH5S^3+ & ceH6S^4+ \
textStable in gas phase? & textYes & textYes & textYes & textYes & textNo \
textApproximate proton affinity (mathrmkJ mol^-1) & 752 & -121 & -1080 & N/A & N/A \
endarray
$$
Notes:
The lower electronegativity and larger size of $ceS$ means its electrons can reach out further and accommodate protons at a larger distance, while reducing repulsions between the nuclei. Thus, in the gas phase, $ceH2S$ is a stronger base than $ceH2O$. The situation is inverted in aqueous solution due to uniquely strong intermolecular interactions (hydrogen bonding) which are much more important for $ceH2O$.
$ceH3S+$ also has an endothermic proton affinity, but it is lower than for $ceH3O+$, and therefore $ceH4S^2+$ is easier to make. Accordingly, $ceH4S^2+$ has been detected in milder (though still superacidic!) conditions than $ceH4O^2+$.
The larger size and lower electronegativity of $ceS$ once again are shown to be important; the hypercoodinate $ceH5S^3+$ appears to exist, while the oxygen analogue doesn't.
$endgroup$
I myself was always confused why $ceH3O^+$ is so well-known and yet almost nobody talks of $ceH4O^2+$. I mean, $ceH3O^+$ still has a lone pair, right? Why can't another proton just latch onto that? Adding to the confusion, $ceH4O^2+$ is very similar to $ceNH4+$, which again is extremely well-known. Even further, the methanium cation $ceCH5+$ exists (admittedly not something you'll find on a shelf), and that doesn't even have an available lone pair!
It is very useful to rephrase the question "why is $ceH4O^2+$ so rare?" into "why won't $ceH3O^+$ accept another proton?". Now we can think of this in terms of an acid-base reaction:
$$ceH3O^+ + H+ -> H4O^2+$$
Yes, that's right. In this reaction $ceH3O^+$ is the base, and $ceH^+$ is the acid. Because solvents can strongly influence the acidity of basicity of dissolved compounds, and because inclusion of solvent makes calculations tremendously more complicated, we will restrict ourselves to the gas phase (hence $ce(g)$ next to all the formulas). This means we will be talking about proton affinities.
Before we get to business, though, let's start with something more familiar:
$$ceH2O(g) + H+(g) -> H3O^+(g)$$
Because this is in the gas phase, we can visualise the process very simply. We start with a lone water molecule in a perfect vacuum. Then, from a very large distance away, a lone proton begins its approach. We can calculate the potential energy of the whole system as a function of the distance between the oxygen atom and the distant proton. We get a graph that looks something like this:
For convenience, we can set the potential energy of the system at 0 when the distance is infinite. At very large distances, the lone proton only very slightly tugs the electrons of the $ceH2O$ molecule, but they attract and the system is slightly stabilised. The attraction gets stronger as the lone proton approaches. However, there is also a repulsive interaction, between the lone proton and the nuclei of the other atoms in the $ceH2O$ molecule. At large distances, the attraction is stronger than the repulsion, but this flips around if the distance is too short. The happy medium is where the extra proton is close enough to dive into the molecule's electron cloud, but not close enough to experience severe repulsions with the other nuclei.
In short, a lone proton from infinity is attracted to a water molecule, and the potential energy decreases up to a critical value, the bond length. The amount of energy lost is the proton affinity: in this scenario, a mole of water molecules reacting with a mole of protons would release approximately $mathrm697 kJ mol^-1$ (values from this table). This reaction is highly exothermic
Alright, now for the next step:
$$ceH3O^+(g) + H+(g) -> H4O^2+(g)$$
This should be similar, right? Actually, no. There is a very important difference between this reaction and the previous one; the reagents now both have a net positive charge. This means there is now a strong additional repulsive force between the two. In fact, the graph above changes completely. Starting from zero potential at infinity, instead of a slow decrease in potential energy, the lone proton has to climb uphill, fighting a net electrostatic repulsion. However, even more interestingly, if the proton does manage to get close enough, the electron cloud can abruptly envelop the additional proton and create a net attraction. The resulting graph now looks more like this:
Very interestingly, the bottom of the "pocket" on the left of the graph (the potential well) can have a higher potential energy than if the lone proton was infinitely far away. This means the reaction is endothermic, but with enough effort, an extra proton can be pushed into the molecule, and it gets trapped in the pocket. Indeed, according to Olah et al., J. Am. Chem. Soc. 1986, 108 (5), pp 1032-1035, the formation of $ceH4O^2+$ in the gas phase was calculated to be endothermic by $mathrm248 kJ mol^-1$ (that is, the proton affinity of $ceH3O^+$ is $mathrm-248 kJ mol^-1$), but once formed, it has a barrier towards decomposition (the activation energy towards release of a proton) of $mathrm184 kJ mol^-1$ (the potential well has a maximum depth of $mathrm184 kJ mol^-1$).
Due to the fact that $ceH4O^2+$ was calculated to form a potential well, it can in principle exist. However, since it is the product of a highly endothermic reaction, unsurprisingly it is very hard to find. The reality in solution phase is more complicated, but its existence has been physically verified (if indirectly).
But why stop here? What about $ceH5O^3+$?
$$ceH4O^2+(g) + H+(g) -> H5O^3+(g)$$
I've run a rough calculation myself using computational chemistry software, and here it seems we really do reach a wall. It appears that $ceH5O^3+$ is an unbound system, which is to say that its potential energy curve has no pocket like the ones above. $ceH5O^3+$ could only ever be made transiently, and it would immediately spit out at least one proton. The reason here really is the massive amount of electrical repulsion, combined with the fact that the electron cloud can't reach out to the distance necessary to accommodate another atom.
You can make your own potential energy graphs here. Note how depending on the combination of parameters, the potential well can lie at negative potential energies (an exothermic reaction) or positive potential energies (an endothermic reaction). Alternatively, the pocket may not exist at all - these are the unbound systems.
EDIT: I've done some calculations of proton affinities/stabilities on several other simple molecules, for comparison. I do not claim the results to be quantitatively correct.
$$
beginarraylllll
textSpecies & ceCH4 & ceCH5+ & ceCH6^2+ & ceCH7^3+ & ceCH8^4+ \
textStable in gas phase? & textYes & textYes & textYes & textYes & textNo \
textApproximate proton affinity (mathrmkJ mol^-1) & 556 & -246 & -1020 & N/A & N/A \
endarray
$$
Notes:
Even without a lone pair, methane ($ceCH4$) protonates very exothermically in the gas phase. This is a testament to the enormous reactivity of a bare proton, and the huge difference it makes to not have push a proton into an already positively-charged ion.
For most of the seemingly hypercoordinate species in these tables (more than four bonds), the excess hydrogen atoms "pair up" such that it can be viewed as a $ceH2$ molecule binding sideways to the central atom. See the methanium link at the start.
$$
beginarraylllll
textSpecies & ceNH3 & ceNH4+ & ceNH5^2+ & ceNH6^3+ \
textStable in gas phase? & textYes & textYes & textYes & textNo \
textApproximate proton affinity (mathrmkJ mol^-1) & 896 & -410 & N/A & N/A \
endarray
$$
Notes:
Even though the first protonation is easier relative to $ceCH4$, the second one is harder. This is likely because increasing the electronegativity of the central atom makes the electron cloud "stiffer", and less accommodating to all those extra protons.
The $ceNH5^2+$ ion, unlike other ions listed here with more than four hydrogens, appears to be a true hypercoordinate species. Del Bene et al. indicate a five-coordinate square pyramidal structure with delocalized nitrogen-hydrogen bonds.
$$
beginarraylllll
textSpecies & ceH2O & ceH3O+ & ceH4O^2+ & ceH5O^3+ \
textStable in gas phase? & textYes & textYes & textYes & textNo \
textApproximate proton affinity (mathrmkJ mol^-1) & 722 & -236 & N/A & N/A \
endarray
$$
Notes:
- The first series which does not accommodate proton hypercoordination.
$ceH3O+$ is easier to protonate than $ceNH4+$, even though oxygen is more electronegative. This is because the $ceH4O^2+$ nicely accommodates all protons, while one of the protons in $ceNH5^2+$ has to fight for its space.
$$
beginarraylllll
textSpecies & ceHF & ceH2F+ & ceH3F^2+ & ceH4F^3+ \
textStable in gas phase? & textYes & textYes & textYes & textNo \
textApproximate proton affinity (mathrmkJ mol^-1) & 501 & -459 & N/A & N/A \
endarray
$$
Notes:
- Even though $ceH3F^2+$ still formally has a lone pair, its electron cloud is now so stiff that it cannot reach out to another proton even at normal bonding distance.
$$
beginarraylllll
textSpecies & ceNe & ceNeH+ & ceNeH2^2+ \
textStable in gas phase? & textYes & textYes & textNo \
textApproximate proton affinity (mathrmkJ mol^-1) & 204 & N/A & N/A \
endarray
$$
Notes:
$ceNe$ is a notoriously unreactive noble gas, but it too will react exothermically with a bare proton in the gas phase.- Depending on the definition of electronegativity used, it is possible to determine an electronegativity for $ceNe$, which turns out to be even higher than $ceF$. Accordingly, its electron cloud is even stiffer.
$$
beginarraylllll
textSpecies & ceH2S & ceH3S+ & ceH4S^2+ & ceH5S^3+ & ceH6S^4+ \
textStable in gas phase? & textYes & textYes & textYes & textYes & textNo \
textApproximate proton affinity (mathrmkJ mol^-1) & 752 & -121 & -1080 & N/A & N/A \
endarray
$$
Notes:
The lower electronegativity and larger size of $ceS$ means its electrons can reach out further and accommodate protons at a larger distance, while reducing repulsions between the nuclei. Thus, in the gas phase, $ceH2S$ is a stronger base than $ceH2O$. The situation is inverted in aqueous solution due to uniquely strong intermolecular interactions (hydrogen bonding) which are much more important for $ceH2O$.
$ceH3S+$ also has an endothermic proton affinity, but it is lower than for $ceH3O+$, and therefore $ceH4S^2+$ is easier to make. Accordingly, $ceH4S^2+$ has been detected in milder (though still superacidic!) conditions than $ceH4O^2+$.
The larger size and lower electronegativity of $ceS$ once again are shown to be important; the hypercoodinate $ceH5S^3+$ appears to exist, while the oxygen analogue doesn't.
edited Apr 5 at 11:44
Oscar Lanzi
16.4k12749
16.4k12749
answered Apr 4 at 3:12


Nicolau Saker NetoNicolau Saker Neto
20k45897
20k45897
1
$begingroup$
@santimirandarp I don't have good graphing software on me right now, but I made something on Desmos. I also put a link to graphs that curious readers may play around with.
$endgroup$
– Nicolau Saker Neto
Apr 4 at 10:18
1
$begingroup$
Unstable by 248 kJ/mol! I smell a monopropellent.
$endgroup$
– Joshua
Apr 4 at 15:43
20
$begingroup$
I've rarely seen this level of investigation in an SE answer. This answer could be turned into a manuscript and published in a reputable journal.
$endgroup$
– WaterMolecule
Apr 4 at 18:42
3
$begingroup$
The "pairing up" of excess hydrogen atoms (beyond 4) may not be entirely accurate. This reference renders the NH5 cation as a square pyramid with multiple delocalized bonds.
$endgroup$
– Oscar Lanzi
Apr 4 at 22:11
1
$begingroup$
@OscarLanzi Huh, interestingly enough that's also what my calculations found, and I overlooked it. It seems to be the only truly hypercoordinate structure out of all the ones I calculated. I was planning on linking the geometries for each structure, but I'll have to do it later. Thanks for the tip, though!
$endgroup$
– Nicolau Saker Neto
Apr 4 at 22:19
|
show 2 more comments
1
$begingroup$
@santimirandarp I don't have good graphing software on me right now, but I made something on Desmos. I also put a link to graphs that curious readers may play around with.
$endgroup$
– Nicolau Saker Neto
Apr 4 at 10:18
1
$begingroup$
Unstable by 248 kJ/mol! I smell a monopropellent.
$endgroup$
– Joshua
Apr 4 at 15:43
20
$begingroup$
I've rarely seen this level of investigation in an SE answer. This answer could be turned into a manuscript and published in a reputable journal.
$endgroup$
– WaterMolecule
Apr 4 at 18:42
3
$begingroup$
The "pairing up" of excess hydrogen atoms (beyond 4) may not be entirely accurate. This reference renders the NH5 cation as a square pyramid with multiple delocalized bonds.
$endgroup$
– Oscar Lanzi
Apr 4 at 22:11
1
$begingroup$
@OscarLanzi Huh, interestingly enough that's also what my calculations found, and I overlooked it. It seems to be the only truly hypercoordinate structure out of all the ones I calculated. I was planning on linking the geometries for each structure, but I'll have to do it later. Thanks for the tip, though!
$endgroup$
– Nicolau Saker Neto
Apr 4 at 22:19
1
1
$begingroup$
@santimirandarp I don't have good graphing software on me right now, but I made something on Desmos. I also put a link to graphs that curious readers may play around with.
$endgroup$
– Nicolau Saker Neto
Apr 4 at 10:18
$begingroup$
@santimirandarp I don't have good graphing software on me right now, but I made something on Desmos. I also put a link to graphs that curious readers may play around with.
$endgroup$
– Nicolau Saker Neto
Apr 4 at 10:18
1
1
$begingroup$
Unstable by 248 kJ/mol! I smell a monopropellent.
$endgroup$
– Joshua
Apr 4 at 15:43
$begingroup$
Unstable by 248 kJ/mol! I smell a monopropellent.
$endgroup$
– Joshua
Apr 4 at 15:43
20
20
$begingroup$
I've rarely seen this level of investigation in an SE answer. This answer could be turned into a manuscript and published in a reputable journal.
$endgroup$
– WaterMolecule
Apr 4 at 18:42
$begingroup$
I've rarely seen this level of investigation in an SE answer. This answer could be turned into a manuscript and published in a reputable journal.
$endgroup$
– WaterMolecule
Apr 4 at 18:42
3
3
$begingroup$
The "pairing up" of excess hydrogen atoms (beyond 4) may not be entirely accurate. This reference renders the NH5 cation as a square pyramid with multiple delocalized bonds.
$endgroup$
– Oscar Lanzi
Apr 4 at 22:11
$begingroup$
The "pairing up" of excess hydrogen atoms (beyond 4) may not be entirely accurate. This reference renders the NH5 cation as a square pyramid with multiple delocalized bonds.
$endgroup$
– Oscar Lanzi
Apr 4 at 22:11
1
1
$begingroup$
@OscarLanzi Huh, interestingly enough that's also what my calculations found, and I overlooked it. It seems to be the only truly hypercoordinate structure out of all the ones I calculated. I was planning on linking the geometries for each structure, but I'll have to do it later. Thanks for the tip, though!
$endgroup$
– Nicolau Saker Neto
Apr 4 at 22:19
$begingroup$
@OscarLanzi Huh, interestingly enough that's also what my calculations found, and I overlooked it. It seems to be the only truly hypercoordinate structure out of all the ones I calculated. I was planning on linking the geometries for each structure, but I'll have to do it later. Thanks for the tip, though!
$endgroup$
– Nicolau Saker Neto
Apr 4 at 22:19
|
show 2 more comments
$begingroup$
In chemistry ask "why" only after you ask "if".
Given a sufficiently strong superacidic medium, $ceH3O^+$ can be protonated to $ceH4O^2+$. Evidence for this reaction, by studying isotopic exchange in a $ceHF + SbF5 +SO2$ solvent, is given here.
$ceH4O^2+$ is, of course, a powerful protic acid, and it would be leveled to something weaker, meaning $ceH3O^+$ and maybe other products, except in superacids as in the above reference.
A subtle difference between $ceH4O^2+$ and $ceSO4^2-$ is the presence of atoms with odd atomic number in the former. An odd atomic number means an odd number of protons, so $ceH4O^2+$ can split off or transfer a component ($ceH^+$) having a single charge and an odd number of odd-proton atoms, without having to break up any electron pairs (no need to form radicals). Hence, unless the environment is suffuciently acidic/nonbasic, this ion could break up relatively easily to singly charged products. $ceSO4^2-$ lacks this option; as this ion has only even atomic number atoms it cannot break up into singly charged species without the unfavorable formation of radicals.
We see a similar phenomenon in the autoionization of solvents like water, ammonia or even dinitrogen tetroxide (p. 217). Solvents are more likely to autoionize if they have odd atomic number atoms (like hydrogen or nitrogen) with which singly charged, nonradical species can form.
$endgroup$
2
$begingroup$
Good point. If you can't break a molecule without making radicals or moving more than one atom (or subunit), you have a good chance it's at least metastable.
$endgroup$
– Karl
Apr 4 at 20:22
add a comment |
$begingroup$
In chemistry ask "why" only after you ask "if".
Given a sufficiently strong superacidic medium, $ceH3O^+$ can be protonated to $ceH4O^2+$. Evidence for this reaction, by studying isotopic exchange in a $ceHF + SbF5 +SO2$ solvent, is given here.
$ceH4O^2+$ is, of course, a powerful protic acid, and it would be leveled to something weaker, meaning $ceH3O^+$ and maybe other products, except in superacids as in the above reference.
A subtle difference between $ceH4O^2+$ and $ceSO4^2-$ is the presence of atoms with odd atomic number in the former. An odd atomic number means an odd number of protons, so $ceH4O^2+$ can split off or transfer a component ($ceH^+$) having a single charge and an odd number of odd-proton atoms, without having to break up any electron pairs (no need to form radicals). Hence, unless the environment is suffuciently acidic/nonbasic, this ion could break up relatively easily to singly charged products. $ceSO4^2-$ lacks this option; as this ion has only even atomic number atoms it cannot break up into singly charged species without the unfavorable formation of radicals.
We see a similar phenomenon in the autoionization of solvents like water, ammonia or even dinitrogen tetroxide (p. 217). Solvents are more likely to autoionize if they have odd atomic number atoms (like hydrogen or nitrogen) with which singly charged, nonradical species can form.
$endgroup$
2
$begingroup$
Good point. If you can't break a molecule without making radicals or moving more than one atom (or subunit), you have a good chance it's at least metastable.
$endgroup$
– Karl
Apr 4 at 20:22
add a comment |
$begingroup$
In chemistry ask "why" only after you ask "if".
Given a sufficiently strong superacidic medium, $ceH3O^+$ can be protonated to $ceH4O^2+$. Evidence for this reaction, by studying isotopic exchange in a $ceHF + SbF5 +SO2$ solvent, is given here.
$ceH4O^2+$ is, of course, a powerful protic acid, and it would be leveled to something weaker, meaning $ceH3O^+$ and maybe other products, except in superacids as in the above reference.
A subtle difference between $ceH4O^2+$ and $ceSO4^2-$ is the presence of atoms with odd atomic number in the former. An odd atomic number means an odd number of protons, so $ceH4O^2+$ can split off or transfer a component ($ceH^+$) having a single charge and an odd number of odd-proton atoms, without having to break up any electron pairs (no need to form radicals). Hence, unless the environment is suffuciently acidic/nonbasic, this ion could break up relatively easily to singly charged products. $ceSO4^2-$ lacks this option; as this ion has only even atomic number atoms it cannot break up into singly charged species without the unfavorable formation of radicals.
We see a similar phenomenon in the autoionization of solvents like water, ammonia or even dinitrogen tetroxide (p. 217). Solvents are more likely to autoionize if they have odd atomic number atoms (like hydrogen or nitrogen) with which singly charged, nonradical species can form.
$endgroup$
In chemistry ask "why" only after you ask "if".
Given a sufficiently strong superacidic medium, $ceH3O^+$ can be protonated to $ceH4O^2+$. Evidence for this reaction, by studying isotopic exchange in a $ceHF + SbF5 +SO2$ solvent, is given here.
$ceH4O^2+$ is, of course, a powerful protic acid, and it would be leveled to something weaker, meaning $ceH3O^+$ and maybe other products, except in superacids as in the above reference.
A subtle difference between $ceH4O^2+$ and $ceSO4^2-$ is the presence of atoms with odd atomic number in the former. An odd atomic number means an odd number of protons, so $ceH4O^2+$ can split off or transfer a component ($ceH^+$) having a single charge and an odd number of odd-proton atoms, without having to break up any electron pairs (no need to form radicals). Hence, unless the environment is suffuciently acidic/nonbasic, this ion could break up relatively easily to singly charged products. $ceSO4^2-$ lacks this option; as this ion has only even atomic number atoms it cannot break up into singly charged species without the unfavorable formation of radicals.
We see a similar phenomenon in the autoionization of solvents like water, ammonia or even dinitrogen tetroxide (p. 217). Solvents are more likely to autoionize if they have odd atomic number atoms (like hydrogen or nitrogen) with which singly charged, nonradical species can form.
edited Apr 4 at 23:23
answered Apr 3 at 23:21
Oscar LanziOscar Lanzi
16.4k12749
16.4k12749
2
$begingroup$
Good point. If you can't break a molecule without making radicals or moving more than one atom (or subunit), you have a good chance it's at least metastable.
$endgroup$
– Karl
Apr 4 at 20:22
add a comment |
2
$begingroup$
Good point. If you can't break a molecule without making radicals or moving more than one atom (or subunit), you have a good chance it's at least metastable.
$endgroup$
– Karl
Apr 4 at 20:22
2
2
$begingroup$
Good point. If you can't break a molecule without making radicals or moving more than one atom (or subunit), you have a good chance it's at least metastable.
$endgroup$
– Karl
Apr 4 at 20:22
$begingroup$
Good point. If you can't break a molecule without making radicals or moving more than one atom (or subunit), you have a good chance it's at least metastable.
$endgroup$
– Karl
Apr 4 at 20:22
add a comment |
$begingroup$
$ceH4O^2+$ would be much smaller than $ceSO4^2-$. The charge density is too high much higher.
The oxygen in water already becomes electron-deficient when it forms $ceH3O+$. In your (not completely, see below) hypothetical $ceH4O^2+$, the additional proton barely finds any electron density to make a covalent-ish bond with.
Whereas for the sulfate ion, you just have to distribute some extra charge over a relatively large molecule.
So $ceH4O^2+$ exists, at least in theory, but it is extremely unstable. It's properties have been detailed (with links to scientific papers) here: What is the Ka of OH- and Kb of H3O+?
$endgroup$
3
$begingroup$
It is quite a valid species, OP is just wondering why it's very difficult to make, even transiently.
$endgroup$
– Mithoron
Apr 3 at 21:25
1
$begingroup$
@Mithoron Are you implying that this particle can exist, at least theoretically and with a very short lifetime, in a bound state? I find that hard to believe, unless of course you have some evidence? (Ok, found it under the link you gave above pubs.acs.org/doi/pdf/10.1021/ja00265a031)
$endgroup$
– Karl
Apr 3 at 21:50
1
$begingroup$
Yeah, it seems it's repulsive, but metastable.
$endgroup$
– Mithoron
Apr 3 at 22:17
add a comment |
$begingroup$
$ceH4O^2+$ would be much smaller than $ceSO4^2-$. The charge density is too high much higher.
The oxygen in water already becomes electron-deficient when it forms $ceH3O+$. In your (not completely, see below) hypothetical $ceH4O^2+$, the additional proton barely finds any electron density to make a covalent-ish bond with.
Whereas for the sulfate ion, you just have to distribute some extra charge over a relatively large molecule.
So $ceH4O^2+$ exists, at least in theory, but it is extremely unstable. It's properties have been detailed (with links to scientific papers) here: What is the Ka of OH- and Kb of H3O+?
$endgroup$
3
$begingroup$
It is quite a valid species, OP is just wondering why it's very difficult to make, even transiently.
$endgroup$
– Mithoron
Apr 3 at 21:25
1
$begingroup$
@Mithoron Are you implying that this particle can exist, at least theoretically and with a very short lifetime, in a bound state? I find that hard to believe, unless of course you have some evidence? (Ok, found it under the link you gave above pubs.acs.org/doi/pdf/10.1021/ja00265a031)
$endgroup$
– Karl
Apr 3 at 21:50
1
$begingroup$
Yeah, it seems it's repulsive, but metastable.
$endgroup$
– Mithoron
Apr 3 at 22:17
add a comment |
$begingroup$
$ceH4O^2+$ would be much smaller than $ceSO4^2-$. The charge density is too high much higher.
The oxygen in water already becomes electron-deficient when it forms $ceH3O+$. In your (not completely, see below) hypothetical $ceH4O^2+$, the additional proton barely finds any electron density to make a covalent-ish bond with.
Whereas for the sulfate ion, you just have to distribute some extra charge over a relatively large molecule.
So $ceH4O^2+$ exists, at least in theory, but it is extremely unstable. It's properties have been detailed (with links to scientific papers) here: What is the Ka of OH- and Kb of H3O+?
$endgroup$
$ceH4O^2+$ would be much smaller than $ceSO4^2-$. The charge density is too high much higher.
The oxygen in water already becomes electron-deficient when it forms $ceH3O+$. In your (not completely, see below) hypothetical $ceH4O^2+$, the additional proton barely finds any electron density to make a covalent-ish bond with.
Whereas for the sulfate ion, you just have to distribute some extra charge over a relatively large molecule.
So $ceH4O^2+$ exists, at least in theory, but it is extremely unstable. It's properties have been detailed (with links to scientific papers) here: What is the Ka of OH- and Kb of H3O+?
edited Apr 4 at 19:45
answered Apr 3 at 18:19
KarlKarl
6,4061435
6,4061435
3
$begingroup$
It is quite a valid species, OP is just wondering why it's very difficult to make, even transiently.
$endgroup$
– Mithoron
Apr 3 at 21:25
1
$begingroup$
@Mithoron Are you implying that this particle can exist, at least theoretically and with a very short lifetime, in a bound state? I find that hard to believe, unless of course you have some evidence? (Ok, found it under the link you gave above pubs.acs.org/doi/pdf/10.1021/ja00265a031)
$endgroup$
– Karl
Apr 3 at 21:50
1
$begingroup$
Yeah, it seems it's repulsive, but metastable.
$endgroup$
– Mithoron
Apr 3 at 22:17
add a comment |
3
$begingroup$
It is quite a valid species, OP is just wondering why it's very difficult to make, even transiently.
$endgroup$
– Mithoron
Apr 3 at 21:25
1
$begingroup$
@Mithoron Are you implying that this particle can exist, at least theoretically and with a very short lifetime, in a bound state? I find that hard to believe, unless of course you have some evidence? (Ok, found it under the link you gave above pubs.acs.org/doi/pdf/10.1021/ja00265a031)
$endgroup$
– Karl
Apr 3 at 21:50
1
$begingroup$
Yeah, it seems it's repulsive, but metastable.
$endgroup$
– Mithoron
Apr 3 at 22:17
3
3
$begingroup$
It is quite a valid species, OP is just wondering why it's very difficult to make, even transiently.
$endgroup$
– Mithoron
Apr 3 at 21:25
$begingroup$
It is quite a valid species, OP is just wondering why it's very difficult to make, even transiently.
$endgroup$
– Mithoron
Apr 3 at 21:25
1
1
$begingroup$
@Mithoron Are you implying that this particle can exist, at least theoretically and with a very short lifetime, in a bound state? I find that hard to believe, unless of course you have some evidence? (Ok, found it under the link you gave above pubs.acs.org/doi/pdf/10.1021/ja00265a031)
$endgroup$
– Karl
Apr 3 at 21:50
$begingroup$
@Mithoron Are you implying that this particle can exist, at least theoretically and with a very short lifetime, in a bound state? I find that hard to believe, unless of course you have some evidence? (Ok, found it under the link you gave above pubs.acs.org/doi/pdf/10.1021/ja00265a031)
$endgroup$
– Karl
Apr 3 at 21:50
1
1
$begingroup$
Yeah, it seems it's repulsive, but metastable.
$endgroup$
– Mithoron
Apr 3 at 22:17
$begingroup$
Yeah, it seems it's repulsive, but metastable.
$endgroup$
– Mithoron
Apr 3 at 22:17
add a comment |
Thanks for contributing an answer to Chemistry Stack Exchange!
- Please be sure to answer the question. Provide details and share your research!
But avoid …
- Asking for help, clarification, or responding to other answers.
- Making statements based on opinion; back them up with references or personal experience.
Use MathJax to format equations. MathJax reference.
To learn more, see our tips on writing great answers.
Sign up or log in
StackExchange.ready(function ()
StackExchange.helpers.onClickDraftSave('#login-link');
);
Sign up using Google
Sign up using Facebook
Sign up using Email and Password
Post as a guest
Required, but never shown
StackExchange.ready(
function ()
StackExchange.openid.initPostLogin('.new-post-login', 'https%3a%2f%2fchemistry.stackexchange.com%2fquestions%2f112087%2fwhy-doesnt-h%25e2%2582%2584o%25c2%25b2%25e2%2581%25ba-exist%23new-answer', 'question_page');
);
Post as a guest
Required, but never shown
Sign up or log in
StackExchange.ready(function ()
StackExchange.helpers.onClickDraftSave('#login-link');
);
Sign up using Google
Sign up using Facebook
Sign up using Email and Password
Post as a guest
Required, but never shown
Sign up or log in
StackExchange.ready(function ()
StackExchange.helpers.onClickDraftSave('#login-link');
);
Sign up using Google
Sign up using Facebook
Sign up using Email and Password
Post as a guest
Required, but never shown
Sign up or log in
StackExchange.ready(function ()
StackExchange.helpers.onClickDraftSave('#login-link');
);
Sign up using Google
Sign up using Facebook
Sign up using Email and Password
Sign up using Google
Sign up using Facebook
Sign up using Email and Password
Post as a guest
Required, but never shown
Required, but never shown
Required, but never shown
Required, but never shown
Required, but never shown
Required, but never shown
Required, but never shown
Required, but never shown
Required, but never shown
3 qRMCwwVU,oe U2UIv7z,OvxjGXL,bg C OoR nC r5 v6FVuIX3nrttXYCKSBvA6Cq nsDN9x1c,PDvnzkaI0E4GHK7 Lg
10
$begingroup$
Note that even $ceH3O+$ is questionable, as some sources prefer existence of $ceH9O4+ $, where each of 3 hydrogen atoms is bound to another $ceH2O$.
$endgroup$
– Poutnik
Apr 3 at 15:43
1
$begingroup$
Yes but isn't it kind of like hydration, where H2O molecules surround the ions , whereas in H3O+ a covalent bond exists between O and H?
$endgroup$
– Sanom Dane
Apr 3 at 15:48
3
$begingroup$
Well, it can be, it could be considered as coordination bonds of 3 H2O ligands. The idea was, that not only the naked $ceH+$ does not exist, but neither the naked $ceH3O+$. pubs.acs.org/doi/abs/10.1021/ic00126a005 A Crystalline [H9O4]+ Hydronium Ion Salt with a Weakly Coordinating Anion
$endgroup$
– Poutnik
Apr 3 at 15:53
2
$begingroup$
I do not doubt $ceH3O+$ exists, I have implied the liquid water context.
$endgroup$
– Poutnik
Apr 3 at 18:26
4
$begingroup$
Related question, though the asker may already be aware.
$endgroup$
– Nicolau Saker Neto
Apr 3 at 20:57